What is the Krebs Cycle?
The Krebs Cycle, which is also widely recognized by its alternative names, the Citric Acid Cycle and the Tricarboxylic Acid (TCA) Cycle, represents a fundamental metabolic pathway that occurs within the mitochondria of eukaryotic cells. This intricate cycle is essential for the conversion of various nutrients, particularly carbohydrates, fats, and proteins, into usable energy, specifically in the form of adenosine triphosphate (ATP). The Krebs Cycle is not merely a series of chemical reactions; it is a critical component of cellular respiration, a process that is indispensable for sustaining life by providing the energy necessary for nearly every biological function that the body undertakes.
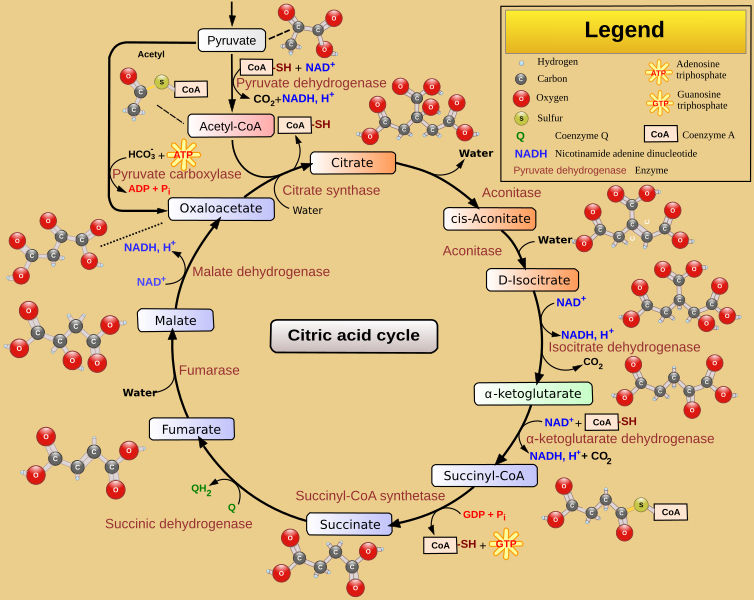
During the Krebs Cycle, acetyl-CoA, derived from the breakdown of glucose, fatty acids, and amino acids, enters the cycle and undergoes a series of transformations. The cycle begins with the condensation of acetyl-CoA with oxaloacetate to form citric acid, a six-carbon compound. As the cycle progresses, citric acid is systematically oxidized and decarboxylated, leading to the release of carbon dioxide and the regeneration of oxaloacetate, which allows the cycle to continue. Throughout this process, high-energy electron carriers, such as NADH and FADH2, are produced. These carriers play a pivotal role in the electron transport chain, where they contribute to the generation of ATP through oxidative phosphorylation.
The significance of the Krebs Cycle extends beyond energy production. It is also a hub for various metabolic pathways, integrating the metabolism of carbohydrates, fats, and proteins. Intermediates generated during the cycle serve as precursors for the biosynthesis of important biomolecules, including amino acids and nucleotides. Additionally, the cycle is regulated by the energy needs of the cell; when energy levels are high, certain enzymes are inhibited, while low energy levels stimulate the cycle to enhance ATP production.
In summary, the Krebs Cycle is an intricate and dynamic metabolic pathway that serves as a cornerstone of cellular respiration. Its ability to efficiently convert nutrients into energy and its role in various biosynthetic processes underscore its importance in maintaining the overall metabolic balance and energy homeostasis within living organisms. Understanding the Krebs Cycle is essential for comprehending how cells harness energy and the biochemical basis of life itself.
Why is the Krebs Cycle Important?
The Krebs Cycle, also known as the citric acid cycle or tricarboxylic acid (TCA) cycle, is a fundamental metabolic pathway that plays a crucial role in cellular respiration and energy production. Its significance extends beyond mere energy generation, encompassing various biochemical processes vital for maintaining cellular health and function. Here are some key reasons why the Krebs Cycle is essential:
Produces the majority of ATP used by cells: The Krebs Cycle is a central component of aerobic respiration, where it facilitates the conversion of carbohydrates, fats, and proteins into usable energy. During this cycle, acetyl-CoA, derived from these macronutrients, is oxidized, leading to the production of ATP through substrate-level phosphorylation. Although the majority of ATP is ultimately generated through oxidative phosphorylation in the Electron Transport Chain (ETC), the Krebs Cycle provides the initial substrates and energy carriers that fuel this process, making it indispensable for cellular energy metabolism.
Generates electron carriers (NADH and FADH2) for the Electron Transport Chain (ETC): One of the critical outputs of the Krebs Cycle is the production of high-energy electron carriers, specifically NADH and FADH2. These molecules are essential as they transport electrons to the ETC, where their energy is harnessed to pump protons across the mitochondrial membrane, creating a proton gradient. This gradient is then used by ATP synthase to generate ATP. Without the continuous production of NADH and FADH2 through the Krebs Cycle, the ETC would lack the necessary electrons to function efficiently, severely impairing ATP synthesis and overall cellular energy supply.
Provides metabolic intermediates for amino acid and fatty acid synthesis: The Krebs Cycle is not only a pathway for energy production but also serves as a hub for various biosynthetic processes. Several intermediates produced during the cycle, such as α-ketoglutarate and oxaloacetate, are precursors for the synthesis of amino acids, which are vital for protein production, cellular repair, and growth. Additionally, citrate, another intermediate, can be transported out of the mitochondria to the cytosol, where it is converted into acetyl-CoA for fatty acid synthesis. This dual role of the Krebs Cycle highlights its importance in both energy metabolism and the synthesis of essential biomolecules.
Supports detoxification pathways in the liver: The Krebs Cycle also plays a significant role in the liver's detoxification processes. Intermediates from the cycle are involved in the urea cycle, which is crucial for the conversion of ammonia, a toxic byproduct of protein metabolism, into urea for excretion. Furthermore, the cycle aids in the metabolism of various xenobiotics, helping to detoxify potentially harmful substances. This function is particularly important for maintaining homeostasis and protecting the body from the accumulation of toxic compounds, underscoring the Krebs Cycle's role in overall metabolic health.
Steps of the Krebs Cycle
The Krebs Cycle consists of eight key enzymatic steps, each catalyzed by specific enzymes. The cycle begins with the combination of acetyl-CoA, which is derived from carbohydrates, fats, and proteins, with oxaloacetate to form citrate. This process is vital for the generation of energy in the form of ATP, as well as for the production of electron carriers NADH and FADH2, which are essential for the electron transport chain. Below is a detailed step-by-step breakdown of the Krebs Cycle:
1. Formation of Citrate
Enzyme: Citrate Synthase
Reaction: Acetyl-CoA + Oxaloacetate → Citrate
In the first step, the enzyme citrate synthase catalyzes the condensation of acetyl-CoA with oxaloacetate, resulting in the formation of citrate. This reaction is a key regulatory point in the cycle and is driven forward by the hydrolysis of the high-energy thioester bond in acetyl-CoA.
2. Formation of Isocitrate
Enzyme: Aconitase
Reaction: Citrate → Isocitrate
The second step involves the conversion of citrate into isocitrate, facilitated by the enzyme aconitase. This process occurs through a two-step mechanism involving the intermediate cis-aconitate, allowing for the rearrangement of the hydroxyl group, which is crucial for the subsequent oxidation reactions.
3. Formation of α-Ketoglutarate
Enzyme: Isocitrate Dehydrogenase
Reaction: Isocitrate → α-Ketoglutarate + CO2 + NADH
In the third step, isocitrate is oxidatively decarboxylated by isocitrate dehydrogenase, resulting in the formation of α-ketoglutarate. This reaction produces carbon dioxide (CO2) as a byproduct and reduces NAD+ to NADH, which carries electrons to the electron transport chain for ATP production.
4. Formation of Succinyl-CoA
Enzyme: α-Ketoglutarate Dehydrogenase
Reaction: α-Ketoglutarate → Succinyl-CoA + CO2 + NADH
The fourth step mirrors the previous decarboxylation. α-Ketoglutarate undergoes further oxidative decarboxylation catalyzed by α-ketoglutarate dehydrogenase, resulting in the formation of succinyl-CoA, another high-energy compound. This step also releases CO2 and produces a second molecule of NADH, highlighting the cycle's role in energy extraction.
5. Formation of Succinate
Enzyme: Succinyl-CoA Synthetase
Reaction: Succinyl-CoA → Succinate + GTP (converted to ATP)
In the fifth step, succinyl-CoA is converted into succinate by the enzyme succinyl-CoA synthetase. This reaction is unique because it is the only step in the Krebs Cycle that directly produces a high-energy phosphate compound, GTP, which can be readily converted to ATP, thus contributing directly to the cell's energy currency.
6. Formation of Fumarate
Enzyme: Succinate Dehydrogenase
Reaction: Succinate → Fumarate + FADH2
The sixth step involves the oxidation of succinate to fumarate, catalyzed by succinate dehydrogenase. During this reaction, FAD (flavin adenine dinucleotide) is reduced to FADH2, another electron carrier that plays a crucial role in the electron transport chain, facilitating further ATP production.
7. Formation of Malate
Enzyme: Fumarase
Reaction: Fumarate → Malate
In the seventh step, fumarate is hydrated to form malate, a reaction catalyzed by the enzyme fumarase. This step is relatively straightforward and serves to prepare malate for the final oxidation step in the cycle.
8. Regeneration of Oxaloacetate
Enzyme: Malate Dehydrogenase
Reaction: Malate → Oxaloacetate + NADH
Finally, in the eighth step, malate is oxidized back to oxaloacetate by malate dehydrogenase. This reaction generates another molecule of NADH, completing the cycle and allowing oxaloacetate to be ready to combine with another acetyl-CoA molecule, thus restarting the Krebs Cycle. The continuous regeneration of oxaloacetate is vital for the cycle’s functionality and efficiency.
The Krebs Cycle then repeats itself, continuously producing energy-rich molecules such as NADH and FADH2, which are crucial for ATP synthesis in the mitochondria. This cycle not only plays an essential role in energy production but also provides intermediates for various biosynthetic pathways, highlighting its significance in cellular metabolism.
Key Nutrients Required for the Krebs Cycle
The efficiency of the Krebs Cycle depends heavily on the presence of specific nutrients that act as cofactors and coenzymes. Here's a list of key nutrients and their functions:
1. B Vitamins
Vitamin B1 (Thiamine): Thiamine plays a crucial role in energy metabolism, particularly as a coenzyme for pyruvate dehydrogenase and α-ketoglutarate dehydrogenase. These enzymes are integral to the conversion of carbohydrates into energy, facilitating the decarboxylation of pyruvate to acetyl-CoA and the oxidative decarboxylation of α-ketoglutarate to succinyl-CoA in the Krebs Cycle. Additionally, thiamine is involved in the synthesis of neurotransmitters, which are essential for proper nerve function, making it vital not just for energy production but also for maintaining a healthy nervous system.
Vitamin B2 (Riboflavin): Riboflavin is an essential precursor to flavin adenine dinucleotide (FAD), a critical coenzyme involved in various metabolic processes. FAD is particularly important for the enzyme succinate dehydrogenase, which plays a dual role in both the Krebs Cycle and the electron transport chain. This vitamin also aids in the metabolism of fats, drugs, and steroids, and acts as an antioxidant, helping to combat oxidative stress in the body. Furthermore, riboflavin is necessary for the maintenance of healthy skin, eyes, and nerve functions, highlighting its multifaceted contributions to overall health.
Vitamin B3 (Niacin): Niacin serves as a precursor to nicotinamide adenine dinucleotide (NAD+), an essential coenzyme that participates in numerous biochemical reactions, including those catalyzed by various dehydrogenase enzymes. NAD+ is pivotal for the conversion of food into energy, as it plays a key role in the Krebs Cycle and in the process of oxidative phosphorylation. Beyond its role in energy metabolism, niacin is also involved in DNA repair and the production of steroid hormones in the adrenal glands. Its importance extends to cardiovascular health, where it can help improve cholesterol levels, thereby reducing the risk of heart disease.
Vitamin B5 (Pantothenic Acid): Pantothenic acid is a vital component of Coenzyme A (CoA), which is critical for the synthesis and oxidation of fatty acids and for the formation of acetyl-CoA, a central metabolite in energy production. Acetyl-CoA serves as a key substrate for the Krebs Cycle, where it is utilized to generate ATP, the energy currency of the cell. In addition to its metabolic functions, vitamin B5 is involved in the synthesis of neurotransmitters and steroid hormones, contributing to the regulation of various physiological processes, including stress responses and mood stabilization.
Vitamin B6 (Pyridoxine): Pyridoxine is essential for amino acid metabolism, acting as a coenzyme in various enzymatic reactions that facilitate the conversion of amino acids into neurotransmitters, such as serotonin and dopamine. This vitamin is vital for the Krebs Cycle, as it supports the transamination processes that feed amino acids into the cycle, influencing energy production. Additionally, vitamin B6 plays a role in the synthesis of hemoglobin, which is necessary for oxygen transport in the blood, and it contributes to immune function and cognitive development, underscoring its importance in maintaining overall health.
Vitamin B7 (Biotin): Biotin is a coenzyme that supports anaplerotic reactions, which are crucial for replenishing intermediates in the Krebs Cycle. By facilitating the carboxylation of pyruvate to form oxaloacetate, biotin helps maintain the cycle's balance and efficiency, ensuring a steady supply of energy. Beyond its metabolic functions, biotin is also known for its role in promoting healthy skin, hair, and nails. It aids in the metabolism of carbohydrates, fats, and proteins, making it an essential nutrient for overall growth and development. Additionally, biotin supports gene regulation and cellular signaling, highlighting its broad impact on health.
2. Magnesium (Mg)
Magnesium (Mg) plays a critical role as a cofactor for several key enzymes involved in the Krebs Cycle. This essential mineral is necessary for the proper functioning of enzymes that facilitate the conversion of carbohydrates, fats, and proteins into energy. Specifically, magnesium is involved in the enzymatic reactions that produce ATP (adenosine triphosphate), the energy currency of the cell. Without adequate magnesium, the efficiency of ATP production can be significantly impaired, leading to reduced energy levels and metabolic dysfunction. Furthermore, magnesium contributes to the stabilization of ATP and is required for the phosphorylation process, which is essential for energy transfer within cells. It is also noteworthy that magnesium deficiency can lead to a cascade of metabolic disturbances, impacting not only energy production but also overall cellular health and function.
3. Iron (Fe)
Iron (Fe) is an essential trace element that plays an integral role in the process of electron transport, which is a crucial component of cellular respiration. Within the Krebs Cycle, iron is particularly involved in the function of succinate dehydrogenase, an enzyme that catalyzes the conversion of succinate to fumarate while simultaneously reducing ubiquinone to ubiquinol. This reaction is pivotal as it links the Krebs Cycle to the Electron Transport Chain (ETC), allowing for the efficient transfer of electrons and the generation of a proton gradient across the mitochondrial membrane. The presence of iron in heme groups and iron-sulfur clusters in various enzymes facilitates the transfer of electrons, which is necessary for ATP synthesis. A deficiency in iron can lead to decreased oxygen transport, impaired energy production, and overall diminished cellular function, underlining its importance in metabolic processes and energy homeostasis.
4. Lipoic Acid
Lipoic acid, a naturally occurring compound, acts as a coenzyme for several critical enzyme complexes, particularly the pyruvate dehydrogenase and α-ketoglutarate dehydrogenase complexes. These complexes are essential for the decarboxylation of pyruvate and α-ketoglutarate, respectively, facilitating the conversion of these substrates into acetyl-CoA and succinyl-CoA. This process is vital for linking glycolysis and the Krebs Cycle, thereby playing a significant role in energy metabolism. Lipoic acid is unique in that it is both water-soluble and fat-soluble, allowing it to function in various cellular environments. Additionally, it possesses antioxidant properties, helping to neutralize free radicals and protect cells from oxidative stress. The presence of lipoic acid is crucial not only for energy production but also for maintaining cellular integrity and function, particularly under conditions of metabolic stress or high energy demand.
5. Coenzyme Q10 (Ubiquinone)
Coenzyme Q10, also known as ubiquinone, plays a pivotal role in the bioenergetic processes of the cell, particularly within the Electron Transport Chain (ETC). Although it is not directly part of the Krebs Cycle, it is essential for the efficient transfer of electrons from the complexes of the ETC to oxygen, which is the final electron acceptor. Coenzyme Q10 functions as a mobile electron carrier that shuttles electrons between the various enzyme complexes, facilitating the generation of a proton gradient across the inner mitochondrial membrane. This gradient is crucial for ATP synthesis via oxidative phosphorylation. Additionally, Coenzyme Q10 has antioxidant properties, helping to protect mitochondrial membranes from oxidative damage and maintaining cellular health. Its levels in the body can decrease with age or certain health conditions, which may lead to reduced energy production and increased oxidative stress, highlighting its importance in both energy metabolism and cellular protection.
Best Dietary Sources to Support the Krebs Cycle
Ensuring your diet contains sufficient amounts of these nutrients can optimize the Krebs Cycle and overall energy metabolism.
Foods Rich in B Vitamins:
Whole grains (brown rice, oats, quinoa)
Leafy greens (spinach, kale)
Legumes (lentils, chickpeas, black beans)
Nuts and seeds (sunflower seeds, almonds)
Eggs and dairy (milk, yogurt, cheese)
Lean meats (chicken, turkey, beef)
Fish (salmon, tuna, sardines)
Foods High in Magnesium:
Pumpkin seeds
Almonds
Dark chocolate (70%+ cacao)
Avocados
Spinach
Iron-Rich Foods:
Red meat (beef, lamb)
Shellfish (clams, mussels)
Tofu
Legumes (lentils, chickpeas)
Fortified cereals
Sources of Lipoic Acid:
Organ meats (liver, kidney)
Spinach
Broccoli
Tomatoes
Sources of Coenzyme Q10:
Fatty fish (mackerel, salmon)
Organ meats (heart, liver)
Nuts and seeds
Final Thoughts: Supporting Energy Metabolism Naturally
The Krebs Cycle is at the core of your body's ability to produce cellular energy. Optimizing this cycle through proper nutrition can enhance athletic performance, cognitive function, and overall vitality.
As a nutritionist, I recommend adopting a balanced, nutrient-dense diet rich in whole foods to ensure your body has all the necessary cofactors to power this metabolic engine. Pair these foods with a healthy lifestyle, including regular exercise and hydration, to fully support cellular energy production.
Comments